Summary
Do bugs suffer? Does a fly caught in a spider's web consciously experience fear and pain? This piece aims to shed some light on that question by presenting quotations and references from a variety of sources. My personal conclusion is that we should give some weight to the possibility of bug suffering, especially until more evidence is available. Thus, considering the 1018 insects that exist at any given time, there is a huge amount of (potential) suffering in nature due to insects alone. We may also want to consider the ways in which humans impact insects, such as through insecticide use, although insecticides could potentially prevent more suffering than they cause if they avert vast numbers of future offspring that would have mostly died, possibly painfully, soon after being born. (Whether insecticides reduce or increase insect suffering on balance seems unclear. And of course, reducing insect habitat permanently would be more humane than simply spraying pesticides.)
See also: "How to Avoid Hurting Insects". One of the easiest tips is to avoid buying silk, since its production boils silk worms alive.
Contents
Suggestions of pain
-
From "Ask An Entomologist: Can an insect percieve its surrounding or feel pain?":
Do insects experience pain? Yes. Well actually, this concept has been disputed, but I think recent evidence suggests that they do experience what is defined as pain.
- References on this page note that substance P, a neurotransmitter causing pain in humans, has been found in fruit flies. This post states that some insects share some mammalian-type neurotransmitters, like serotonin, dopamine, and acetylcholine. Earthworms have endorphins.
-
From Joan Dunayer's Speciesism (p. 128):
In any case, abundant evidence indicates that all invertebrates with a brain can experience pain. Like vertebrates, numerous invertebrates produce natural opiates and substance P. These animals include crustaceans (e.g., crabs, lobsters, and shrimps), insects (e.g., fruit flies locusts, and cockroaches), and mollusks (e.g., octopuses, squids, and snails).
However, endogenous opioids seem to play roles apart from mediating pain processing, such as reproduction-cycle regulation, midgut function, and movement.
Joan Dunayer continues:
Also, crustaceans, insects, and mollusks show less reaction to a noxious stimulus when they receive morphine. For example, morphine reduces the reaction of mantis shrimps to electric shock, praying mantises to electric shock, and land snails to a hot surface.
One example study is "Morphine analgesia, tolerance and addiction in the cricket Pteronemobius sp. (Orthoptera, Insecta)," which found reduced escape reaction times by crickets from a heated box when they were injected with morphine.
-
Thomas Eisner and Scott Camazine, "Spider leg autotomy induced by prey venom injection: An adaptive response to 'pain'?":
Field observations showed orb-weaving spiders (Argiope spp.) to undergo leg autotomy if they are stung in a leg by venomous insect prey (Phymata fasciata). The response occurs within seconds, before the venom can take lethal action by spread to the body of the spiders. Autotomy is induced also by honeybee venom and wasp venom, as well as by several venom components (serotonin, histamine, phospholipase A2, melittin) known to be responsible for the pain characteristically elicited by venom injection in humans. The sensing mechanism by which spiders detect injected harmful chemicals such as venoms therefore may be fundamentally similar to the one in humans that is coupled with the perception of pain.
Nociception vs. pain
- From Jane A. Smith's "A Question of Pain in Invertebrates":
Invertebrates, it seems, exhibit nociceptive responses analogous to those shown by vertebrates. They can detect and respond to noxious stimuli, and in some cases, these responses can be modified by opioid substances. However, in humans, at least, there is a distinction to be made between the "registering" of a noxious stimulus and the "experience" of pain. In humans, pain "may be seen as the response of the whole awake conscious organism to noxious stimuli, seated.., at the highest levels in the central nervous system, involving emotional and other psychological components" (Iggo, 1984). Experiments on decorticate mammals have shown that complex, though stereotyped, motor responses to noxious stimuli may occur in the absence of consciousness and, therefore, of pain (Iggo, 1984). Thus, it is possible that invertebrates' responses to noxious stimuli (and modifications of these responses) could be simple reflexes, occurring without the animals being aware of experiencing something unpleasant, that is, without "suffering" something akin to what humans call pain.
[...] What evidence might help in distinguishing between nociceptive "responsiveness" and the perception of pain? [...]
In mammals, responses to painful stimuli often persist beyond a simple reflex withdrawal, so that, for example, the animals may become immobile, limp or "guard" the affected part, show aggression when approached, reduce or stop feeding and drinking, and show decreased sexual activity (Morton and Griffiths, 1985). The animals may also learn in the future to avoid situations similar to the one in which the pain occurred. Such responses, while not proof that the animals have experienced pain, can indicate that something more than a simple nociceptive reflex is involved. Together, they may help the animal to recover from damage caused by the painful event and avoid being harmed in the future.
Learning and intelligence
-
A 1986 paper, "Invertebrate Learning and Memory: From Behavior to Molecules," reviewed studies on a number of invertebrates, including bees, slugs, molluscs, snails, leeches, locusts, and fruit flies. The conclusion included the following remarks (pp. 473-76):
The progress achieved over the last 10-15 years in studying a wide variety of forms of learning in simple invertebrate animals is quite striking. There is now no question, for example, that associative learning is a common capacity in several invertebrate species. In fact, the higher-order features of learning seen in some invertebrates (notably bees and Limax) rivals that commonly observed in such star performers in the vertebrate laboratory as pigeons, rats, and rabbits.
[... W]e have reason to hope that the distinction between vertebrate and invertebrate learning and memory is one that will diminish as our understanding of underlying mechanisms increases.
- Perry et al. (2013), p. 570: "Besides feats of navigation, some invertebrates have shown other forms of complex learning. One well-studied example is contextual learning in which an animal must learn that the value of a stimulus depends on other (contextual) conditions[...]. Crickets and cockroaches can learn that different and conflicting odor–food associations apply under different light conditions. Ants, bumblebees, and honeybees have all displayed contextual learning using specific places, direction of flight, time of day, distinct odors, or sides of the body as contexts."
-
Georgia J. Mason, "Invertebrate welfare: where is the real evidence for conscious affective states?":
[...] jumping spiders (Portia spp.) plan routes towards their prey [10]; and hermit crabs (Pagurus berhnardus) show evidence of motivational trade-offs during shell choice [11]. Furthermore, if their brains are implanted with electrodes, garden snails (Helix aspersa) will learn to displace a lever, an action new to their behavioural repertoire, to stimulate those neural regions involved in sexual behaviour [12]. None of these represent concrete evidence of conscious emotion, but they at least suggest that if cephalopods are to now be protected across Europe, then arachnids, decapod crustaceans and gastropods should be too.
"Emotion"
-
"For stressed bees, the glass is half empty":
"We have shown that the emotional responses of bees to an aversive event are more similar to those of humans than previously thought," said Geraldine Wright of Newcastle University. "Bees stressed by a simulated predator attack exhibit pessimism mirroring that seen in depressed and anxious people." [...]
But, they say, that isn't the same as saying that bees consciously experience emotions in the way that we do. On that point, the jury is still out.
"Consciousness"
- The summary of The Ancient Origins of Consciousness reports that the authors "find that arthropods (including insects and probably crustaceans) and cephalopods (including the octopus) meet many of the criteria for consciousness."
-
In response to Edge's World Question Center 2005 topic, "What Do You Believe Is True Even Though You Cannot Prove It?", Alun Anderson, Editor-in-Chief at New Scientist, made the following remarks:
Strangely, I believe that cockroaches are conscious. [Moreover, ...] I believe that many quite simple animals are conscious, including more attractive beasts like bees and butterflies.
[... What I mean by consciousness is] the feeling of "seeing" the world and its associations. For the bee, it is the feeling of being a bee. I don't mean that a bee is self-conscious or spends time thinking about itself. But of course the problem of why the bee has its own "feeling" is the same incomprehensible "hard problem" of why the activity of our nervous system gives rise to our own "feelings."
But at least the bee's world is very visual and capable of being imagined. Some creatures live in sensory worlds that are much harder to access. Spiders that hunt at night live in a world dominated by the detection of faint vibration and of the tiniest flows of air that allow them to see fly passing by in pitch darkness. Sensory hairs that cover their body give them a sensitivity to touch far more finely grained than we can possibly feel through our own skin.
[...] And as for the cockroaches, they are a little more human than the spiders. Like the owners of the New York apartments who detest them, they suffer from stress and can die from it, even without injury. They are also hierarchical and know their little territories well. When they are running for it, think twice before crushing out another world.
-
From a 2007 Discover Magazine article, "Consciousness in a Cockroach":
To Nicholas Strausfeld, a tiny brain is a beautiful thing. Over his 35-year career, the neurobiologist at the University of Arizona at Tucson has probed the minute brain structures of cockroaches, water bugs, velvet worms, brine shrimp, and dozens of other invertebrates. [...] From this tedious analysis Strausfeld concludes that insects possess "the most sophisticated brains on this planet."
Strausfeld and his students are not alone in their devotion. Bruno van Swinderen, a researcher at the Neurosciences Institute (NSI) in San Diego, finds hints of higher cognitive functions in insects—clues to what one scientific journal called "the remote roots of consciousness."
"Many people would pooh-pooh the notion of insects having brains that are in any way comparable to those of primates," Strausfeld adds. "But one has to think of the principles underlying how you put a brain together, and those principles are likely to be universal." [...]
"Attention," says van Swinderen, "is a whole-brain phenomenon. A thing is not purely visual, not purely olfactory. It's a binding together of different parts that for us signify one thing. Why couldn't the fly's mechanism [of attention] be directed to a succession of its memories?" he asks. "That, to me, is just a short hop, skip, and a jump away from what might be consciousness."
In the article, Christof Koch adds:
We have literally no idea at what level of brain complexity consciousness stops. Most people say, 'For heaven's sake, a bug isn't conscious.' But how do we know? We're not sure anymore. I don't kill bugs needlessly anymore. [...]
Probably what consciousness requires is a sufficiently complicated system with massive feedback. Insects have that. If you look at the mushroom bodies, they're massively parallel and have feedback.
Koch tries to avoid stepping on insects when walking.
- Goldman (2016) quotes Antonio Damasio as saying: "Feeling implies the presence of a mind and a mental experience, [or] consciousness. I have every reason to believe that invertebrates not only have emotions but also the possibility of feeling those emotions."
Sleep-like states
- "Neuronal correlates of sleep, wakefulness and arousal in a diurnal insect" (1983) reports that forager bees display neuronal oscillations similar to sleep-wake patterns in mammals and birds.
- "Do insects sleep?" discusses further examples of insect cycles of activity and rest. That said, circadian rhythms are present even in plants, fungi, and cyanobacteria, so this fact alone is not conclusive.
- "Do Bugs Sleep? Why They're Surprisingly Similar to People"
Non-stereotyped behavior
-
In his 1984 Animal Thinking, Donald Griffin presents complex behaviors on the part of various species of insects that he feels suggest consciousness. He concludes chapter 5 with the remark (p. 116):
Explaining instinctive behavior in terms of conscious efforts to match neural templates may be more parsimonious than postulating a complete set of specifications for motor actions that will produce the characteristic structure under all probable conditions. Conscious efforts to match a template may be more economical and efficient. [... Of course] it is not necessary to suppose that animals [including insects] are consciously aware of all their neural templates; perhaps only a few are important enough that the animal thinks consciously about them and considers alternative ways of realizing them.
On p. 105, Griffin elaborates an example:
The workers of leaf-cutter ants are tiny creatures, and their entire central nervous system is less than a millimeter in diameter. Even such a miniature brain contains many thousands of neurons, but ants must do many other things besides gathering leaves and tending fungus gardens. Can the genetic instructions stored in such a diminutive central nervous system prescribe all of the detailed motor actions carried out by one of these ants? Or is it more plausible to suppose that their DNA programs the development of simple generalizations such as "Search for juicy green leaves" or "Nibble away bits of fungus that do not smell right," rather than specifying every flexion and extension of all six appendages?
Page 111 gives an example with spiders:
W. S. Bristowe (1976) describes how orb-weaving spiders sometimes vary their stereotyped behavior in dealing with small insects caught in their webs. If an experimenter holds a struggling fly with forceps close to such a spider, she omits the earlier stages of normal behavior (running along the web to reach the fly) and bites it immediately. If the fly is already dead, she wraps it in silk without biting it first. In constructing their elaborate webs, spiders are often said to follow a rigid series of behavior patterns which are presumably instinctive since a female spinning her first web does so almost perfectly. But she will make some alterations in structure when the surrounding vegetation or the space to be spanned is irregular. Bristowe describes how a spider whose web is ordinarily symmetrical builds a highly asymmetrical web when the opening between leaves makes such a shape appropriate. At the web's hub from which strands of silk radiate out to the surrounding vegetation, the spider ordinarily leaves a hole so she can quickly move from one side of the web to the other when an insect strikes it. In one web this hole, instead of being at the center, was close to one edge of the opening between the leaves of a lilac bush, and the strands formed a semicircle instead of a circle.
Many ethologists dismiss variability in structures such as spider webs as meaningless "noise" in a basically invariant system and deny that a spider could consciously adjust the structure of her web according to the shape of the available opening. But the end results are so efficiently adapted to their function of catching small flying insects that it seems possible that spiders anticipate the likely results of their web spinning.
- This video shows a bee pulling a nail out of a wall in order to get inside the hole that the nail was filling. The bee pulls the nail out bit by bit, seeming to have a sense of what it's trying to accomplish. As the nail is almost out, the bee seems to brace itself for flight, as if it knows that the nail will fall. Of course, this is my interpretation of the bee's behavior, but at least it seems clear that this isn't an inflexible, pre-programmed routine.
Cognitive generalization
Menzel and Giurfa (1999), p. 718:
Liu et al. report their results on visual learning by Drosophila and its underlying neuronal substrate. They show that individual flies can do quite complex tasks. The authors first conditioned flies to associate visual patterns (the ‘conditioned’ stimulus) with the presence or absence of heat (the ‘unconditioned’ stimulus). The idea is that the animals should fly towards the appropriate patterns to avoid dangerous levels of heat. Their behaviour is ‘operant’, because the flight course that they choose determines delivery of the heat. The experiments were done under particular illumination conditions, which form part of the general ‘context’ in which the associations are established.
The authors next showed that the flies could ‘generalize’ this trained response to several other, different environmental contexts. As contexts are ill-defined stimuli, comprising individual features from many modalities, the change in context that the authors introduced was a change in the illumination between training and test: between white and monochromatic broadband light; between two monochromatic broad-band lights; and between constant white light and white light interspersed with ‘dark flashes’ (light is switched off for 200 ms). These changes did not affect the performance of the flies. These results show that context generalization — rather than context specificity — guides the insect’s learning. But when the authors impaired the fly’s normal brain function by eliminating the mushroom bodies (a central brain structure), they found that retention of the trained pattern was strictly bound to the context during learning, and that the flies did not generalize to other contexts.
From "Exploring Consciousness through the Study of Bees" by Christof Koch:
Insects, in particular, were long thought to be simple, reflexive creatures with hardwired instinctual behaviors. No more. Consider the amazing capabilities of the honeybee, Apis mellifera. [...]
In humans, the short-term storage of symbolic information—as when you enter an acquaintance's phone number into your iPhone's memory—is associated with conscious processing. Can bees remember task-relevant information? The gold standard for evaluating working memory is the delayed matching-to-sample (DMTS) paradigm. The subject looks at a picture for a few seconds. The test image then disappears for five or 10 seconds. Subsequently, two pictures are shown next to each other, and the animal has to choose, by pushing a lever or moving its eyes, which of the two images was the test picture. This test can be carried out correctly only if the animal remembers the image. A more complex version, the delayed nonmatching-to-sample (DNMTS) task, requires one additional processing step: choosing the opposite image from the one previously shown.
Although bees can't be expected to push levers, they can be trained to take either the left or the right exit inside a cylinder modified for the DMTS test. A color disk serves as a cue at the entrance of the maze, so that the bee sees it before entering. Once within the maze, the bee has to choose the arm displaying the color that matches (DMTS) or differs from (DNMTS) the color at the entrance. Bees perform both tasks well. They even generalize to a situation they have never previously encountered. That is, once they've been trained with colors, they "get it" and can now follow a trail of vertical stripes if a disk with vertical gratings is left at the entrance of the maze. These experiments tell us that bees have learned an abstract relation (sameness in DMTS, difference in DNMTS) irrespective of the physical nature of the stimuli. The generalization to novel stimuli can even occur from odors to colors. [...]
Although these experiments do not tell us that bees are conscious, they caution us that we have no principled reason at this point to reject this assertion.
Neural oscillations
Prieto-Godino and de Polavieja (2010):
Electroencephalogram and local field potential (LFP) oscillations generally indicate periodic coherent synchronization of neuronal assemblies [1]–[3]. Oscillations have been found in systems as disparate as mollusks [2], moths [4], locusts [5], [6], rats and mice [7], [8], suggesting a fundamental role in computations carried out during higher-order processing. Oscillatory and synchronized activities in the mammalian brain have been correlated with distinct behavioural states or the execution of complex cognitive tasks, and are proposed to participate in the ‘binding’ of individual features into more complex percepts [3], [9]–[11]. Similar oscillations have been observed in LFP recordings from the first and second relay centers for olfactory information in insects. [...] However it is not known whether and how the oscillations observed in insect sensory systems and the ones recorded from mammals during the execution of complex cognitive tasks are functionally and computationally related. A first step in this direction is to find out whether oscillations occur in higher brain structures of insects during the performance of complex cognitive tasks, involving processes such as selective attention [14], contextual generalization [15], or formation of ‘sameness’ and ‘difference’ concepts [16]. Recently two reports have correlated in flies LFP oscillatory activity recorded centrally in the brain with different behavioural states, as it happens in mammals [17], [18]. These studies found that conspicuousness of different visual objects modulates the oscillatory activity recorded from central brain structures in the 20–30 Hz range. [...]
The fruit fly's LFP responses share several key features with physiological correlates in the 40–60 Hz range of visual selective attention in monkeys and humans [31]. For example, amplitude increases with salience, which can be increased by either an unconditioned stimuli or by novelty. [32]. Furthermore, a recent study in rats reported that, during difficult odour discrimination tasks, strong synchronous oscillations appeared in the olfactory bulb field potential. These oscillations were not present when the task was easy and could reflect an increased attention [33]. Therefore in flies, as it has been suggested before in mammals [31], neural synchronization may be a common neural mechanism involved in complex cognitive tasks like arousal, perceptual integration, and attentional selection. Our findings in the olfactory system, together with the findings of others in visual attention [18], indicate that despite the fruit fly's lack of neuroanatomical homology with primates, Drosophila might have analogous mechanisms of establishing salience and directing selective attention to its environment.
Social behavior
-
In his 1987 piece, "The moral standing of insects and the ethics of extinction," Professor Jeffrey Lockwood, an entomologist and philosopher, summarizes arguments for self-awareness based on social interaction:
Another theoretical consideration of insect consciousness, is an extension of the work by Humphrey (1978) (who derived his work from that of Jolly (1966)) on the evolution of societies (Griffin 1984). The basic concept states that a critical step in the evolution of animal societies is the establishment of efficient interactions, and these interactions depend on group members' abilities to understand each others' thoughts, intentions, and feelings. Therefore, social insects must correctly judge the frame of mind, as it were, of one another. [...]
Social insects behave so as to meet the communicated needs of the colony. One can construct a system which awkwardly explains social interaction such as food begging and tropholaxis or behaviors such as grooming, without including self-awareness. However, few would argue that social insects, and probably all insects, demonstrate an awareness of outside events; they behave according to environmental conditions and, as discussed earlier, they demonstrate the ability to communicate information about these conditions. Allowing that an insect has awareness of external events but does not have self-awareness is somewhat ridiculous--it is rather implausible to contend that through sensory mechanisms an insect is aware of the environment, other insects, and the needs of conspecifics but through some neural blockage, the same insect is selectively unconscious of sensory input about itself.
- "The Evolution of Social Behavior in Insects and Arachnids", edited by Jae C. Choe, Bernard J. Crespi:
'Social' insects and arachnids exhibit forms of complex behaviour that involve cooperation in building a nest, defending against attackers or rearing offspring. [... Some chapters from this book] are as follows:
[...]
Post-ovulation parental investment and parental care in cockroaches
The spectrum of eusociality in termites
Maternal care in the Hemiptera: ancestry, alternatives and current adaptive value
Evolution of parental care in the giant water bugs (Heteroptera: Bolostomatidae)
The evolution of sociality in aphids: a clone's eye view
Ecology and evolution of social behaviour among Australian gall thrips
Interactions among males, females and offspring in bark and ambrosia beetles: the significance of living in holes for the evolution of social behaviour
[...]
Morphologically 'primitive' ants: comparative review of social characters, and the importance of queen-worker dimorphism
Social conflict and cooperation among founding queens in ants (Hymenoptera: Formicidae)
Social evolution in the lepidoptera: ecological context and communication in larval societies
Sociality and kin selection in Acari
Colonial web-building spiders: balancing the costs and benefits of group living
-
From the abstract of Lihoreau M, Brepson L, Rivault C, "The weight of the clan: even in insects, social isolation can induce a behavioural syndrome":
Here we report that gregarious cockroaches (Blattella germanica) reared in isolation showed (i) stronger exploration-avoidance, (ii) reduced foraging activity, (iii) reduced willingness to interact socially, and (iv) reduced ability to assess mating partner quality than conspecifics reared in groups. We demonstrate the occurrence of a behavioural syndrome induced by social isolation, similar to syndromes described in vertebrates, revealing the importance of social interactions and group-living in this non-eusocial insect species.
-
"Why cockroaches need their friends" by Matt Walker:
Scientists are discovering that these supposedly crude, and creepy automatons are much more sophisticated than we thought.
By unveiling the secret lives of these insects, they are finding out that cockroaches are actually highly social creatures; they recognise members of their own families, with different generations of the same families living together.
Cockroaches do not like to be left alone, and suffer ill health when they are.
And they form closely bonded, egalitarian societies, based on social structures and rules. Communities of cockroaches are even capable of making collective decisions for the greater good. [...]
Cockroaches that do not hang out with one another suffer "isolation syndromes". For example, young German and American cockroaches left alone take longer to moult into new larger forms and eventually become adults.
Their later behaviour is also severely affected; young isolated cockroaches find it harder to join a community and mate later in life.
Young cockroaches, it seems, need to be around and in constant physical contact with one another to properly develop.
What about tissue damage?
See also: "Which Stimuli Are Painful to Invertebrates?"
-
As Eisemann et al. note in "Do insects feel pain? A biological view" (1984), many insects lack nociceptors and so perhaps would not find tissue damage to be aversive. The authors add:
No example is known to us of an insect showing protective behavior towards injured parts, such as by limping after leg injury or declining to feed or mate because of general abdominal injuries. On the contrary, our experience has been that insects will continue with normal activities even after severe injury or removal of body parts.
-
That said, according to Wikipedia's article on nociceptors:
Nociception has been documented in non-mammalian animals, including fish[9] and a wide range of invertebrates, including leeches,[10] nematode worms,[11] sea slugs,[12] and fruit flies.[13] Although these neurons may have different pathways and relationships to the central nervous system than mammalian nociceptors, nociceptive neurons in non-mammals often fire in response to similar stimuli as mammals, such as high temperature (40 degrees C or more), low pH, capsaicin, and tissue damage.
The Wikipedia article on Pain in invertebrates explains more:
The first invertebrate in which a nociceptive cell was identified was the medicinal leech, Hirudo medicinalis, which has the characteristic segmented body of an Annelida, each segment possessing a ganglion containing the T (touch), P (pressure) and N (noxious) cells.[17] Later studies on the responses of leech neurones to mechanical, chemical and thermal stimulation motivated researchers to write "These properties are typical of mammalian polymodal nociceptors".[4]
There have been numerous studies of learning and memory using nociceptors in the sea hare, Aplysia.[18][19][20] Many of these have focused on mechanosensory neurons innervating the siphon and having their somata (bulbous end) in the abdominal ganglion (LE cells). These LE cells display increasing discharge to increasing pressures, with maximal activation by crushing or tearing stimuli that cause tissue injury. Therefore, they satisfy accepted definitions of nociceptors. They also show similarities to vertebrate Aδ nociceptors, including a property apparently unique (among primary afferents) to nociceptors—sensitization by noxious stimulation. Either pinching or pinning the siphon decreased the threshold of the LE cells firing and enhanced soma excitability.[21]
-
Sonetti et al. (1999) found that a snail species (Planorbarius corneus) and a mussel species (Mytilus galloprovincialis) showed increased levels of endogenous morphine following tissue damage. The animals were damaged as follows (p. 138):
[in] a set of 20 adult specimens of P. corneus, a cut of few millimeters was made in the foot edge with fine scissors. After this surgical incision, that never caused death, the animals were sacrificed 24 and 48 h later. [...] A group of 20 mussels, M. galloprovincialis, were traumatized by cutting with a fine lancet the shell posterior adductor muscle. All animals survived this treatment for up to 24 and 48 h.
Sonetti et al. (1999) conclude (pp. 145-46):
Taken together, the data demonstrates that endogenous morphine is present in specific tissues of these animals and appears to be involved in the response to physical trauma.
The biochemical data obtained from Planorbarius are in accordance with those obtained from M. edulis by Stefano et al. [24,25]. They also detected, between 24 and 48 h, the highest level of morphine in the hemolymph and the pedal ganglia following stressful situation [24,25]. In the present study traumatized snails had significant morphine increases in the ganglia, hemolymph, aorta, immune cells and foot, supporting the idea that morphine may play multiple roles inside nervous, immune and vascular systems. [...]
In conclusion, invertebrate neural and immune cells appear to contain morphinergic signaling processes. Based on past studies, we surmise this molecule may serve to calm or terminate the state of alertness and activation of immune and neural cells created after neural stimulation and/or trauma [17,23,25]. Furthermore, morphinergic signaling appears to resemble classical chemical messenger systems that are referred to as neurotransmitter, neurohormonal, as well as neuro-immune and neuro-vascular. In this regard, it also appears that morphinergic-signaling processes evolved earlier than formerly envisioned.
- This page reports: "Macrobrachium americanum prawns treated with lignocaine (a local anaesthetic in mammals) prior to eyestalk ablation show less rubbing, flicking and sheltering than those not given the anaesthetic.[7]" While prawns are not insects, they are invertebrates. Like prawns, some terrestrial "bugs", such as woodlice, are malacostracan crustaceans.
-
Moreover, not all forms of suffering are due to direct tissue damage. Insects are still motivated to avoid certain things and seek others. Perhaps they can feel the agony of starvation, or dehydration, or internal organ failure? Indeed, insects have plenty of other sense organs, as the Wikipedia entry on insect physiology explains:
Chemical senses include the use of chemoreceptors, related to taste and smell, affecting mating, habitat selection, feeding and parasite-host relationships. [...] Chemoreceptor sensitivity related to smell in some substances, is very high and some insects can detect particular odours that are at low concentrations miles from their original source (Triplehorn & Johnson, 2005).
Mechanical senses provide the insect with information that may direct orientation, general movement, flight from enemies, reproduction and feeding and are elicited from the sense organs that are sensitive to mechanical stimuli such as pressure, touch and vibration (Triplehorn & Johnson, 2005). [...]
Pressure on the body wall or strain gauges are detected by the campiniform sensilla and internal stretch receptors sense muscle distension and digestive system stretching (McGavin 2001; Triplehorn & Johnson, 2005,). [...]
A number of insects have temperature and humidity sensors (McGavin, 2001)
-
In her 2001 "Animal Suffering: An Invertebrate Perspective," Jennifer A. Mather notes:
the physiological systems that control responses to what we call pain mostly are universal across the animal kingdom, and snails often are used as models for such responses. Can we treat them as having sensations that resemble ours without being concerned for their welfare when they do?
Still, it is less easy to take that leap of faith and presume parallels with how you feel when the animal concerned is completely unlike you. Insects, for instance, can walk normally with a couple broken-off legs and survive with apparent unconcern as a parasite is eating them up inside, when presumably we would be in excruciating pain. Does that mean they cannot feel pain? I asked a friend who works with ants what she thought about this apparent inability to feel the pain we do. She said that she spilled a drop of acetone on an ant by accident one day and that it had recoiled and tried to wipe the substance off its abdomen. Maybe it is still pain, just responding to different stimuli. Alternately, maybe it is just an automatic grooming reaction. Because nuclear radiation can kill us without our feeling a thing, humans too do not always respond with pain to possible tissue destruction.
-
Lauritz S. Sømme concludes a report to Norwegian Scientific Committee for Food Safety on "Sentience and Pain in Invertebrates" with this statement about insect sentience:
The nervous system and senses of insects appear to be better developed than in crustaceans since an active life on land may be more demanding. With the great diversity of insects, there are great differences in the organization of the central nervous system and senses. In general, insects are equipped with numerous sense organs. The brain is particularly well developed in social insects, and the size of certain neural centers can be correlated with learning capacity. Learning is also known from many solitary species of insects. Insects do not react to damage of their bodies, but may show strong reflexes to constraint. With our present knowledge, it is usually concluded that insects cannot feel pain. Still, doubts have been raised. Among invertebrates, social insects represent a high level of cognition, and their welfare should be considered during handling.
Benefit of the doubt?
From entomologist Jeffrey Lockwood, "Do bugs feel pain?":
So, given that we can't be sure whether insects experience pain, how should we treat these creatures? When I was teaching insect anatomy and physiology I insisted that the students anesthetized insects before conducting experiments that we would expect to inflict pain on a mouse. [...]
[One reason is] it seems ethically obligatory to guard against the possibility that insects feel pain. If we use anesthetic and it turns out that insects don't experience pain, the material cost of our mistake is very low [...]. However, if we don't use anesthetic and it turns out that the insects were in agony, then the moral cost of our mistake is quite high.
In general I agree with this sentiment. As a matter of detail, I would not use exactly this "precautionary principle" approach of giving insects the benefit of the doubt. I would instead multiply possible insect suffering by a probability of sentience; this makes the ethical tradeoffs between insects, which may or may not suffer, more fair against animals we know can suffer. However, given that insects have a probability of sentience that isn't too small, their potential suffering still tends to dominate calculations even when multiplied by 50%, or 10%, or even 1%. My own probability for sentience is ~40%.a Considering how easy it is to avoid causing harm to so many insects, on a macro scale and even in our daily lives, any reasonable sentience probability will imply significant consequences for our actions.
Further reading
- I highly recommend Section 2.1 - 2.4, pages 15-36 (PDF pages 62-84), of "Aspects of the biology and welfare of animals used for experimental and other scientific purposes," a lengthy document summarized here. In particular, section 2.3 is organized in a similar fashion to the current document. The information presented is too much to summarize, so I encourage readers to take a look at the original source.
- Another excellent review article is Jane A. Smith's "A Question of Pain in Invertebrates."
- A multi-author discussion of insect consciousness in the journal Animal Sentience.
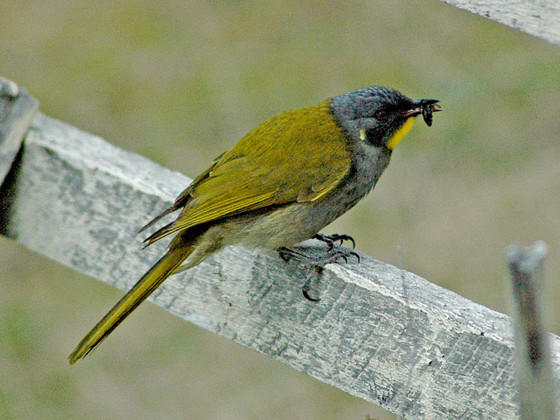
Footnotes
-
I now don't believe that whether insects are sentient is an objective factual question, so talking about probabilities isn't quite accurate. Fundamentally, whether we care about insects is a moral choice. However, facts are very relevant in informing us about (a) what abilities insects actually have and (b) what types of abilities in humans correspond to conscious emotion. For instance, we might have thought that a given cognitive trait was important for human emotional experience but later learn that it's completely irrelevant, and in that case, the fact that insects have it would not be as pertinent as we had presumed. And the same could be true in the opposite direction. So one way to continue using probabilities is to ask "How likely would I be to care about insects if I learned and thought more about the topic for a long time?"
Beyond this, I think sentience should be seen to come in gradations, in which case the question is less whether insects matter or not in a binary fashion but how much they matter. I think it's very likely I would care to some degree about insects upon further reflection, but whether I would care a little or a lot remains uncertain. At the moment I would guess that I value preventing one dog from suffering at around the same as preventing ~100 insect from suffering in an analogous way. This assessment is likely to change over time.