Summary
The net impact of eutrophication on aggregate invertebrate populations in freshwater lakes and rivers is unclear, but evidence points toward greater nutrient inputs causing greater total metazoan zooplankton populations (but reduced mayfly populations). I wonder if these trends depend a lot on whether and how anoxic the lake is.
Note: This page is a work in progress and isn't yet comprehensive.
Contents
Introduction
In many cases, human impacts on the environment reduce the net primary productivity (NPP) of affected regions. In general, this can be expected to lower total animal populations as well, since animals feed (directly and indirectly) on plant biomass.
Eutrophication is a rare situation where human activity substantially increases NPP. Thus, a priori, we would expect eutrophication to increase animal populations. However, in lakes that become sufficiently anoxic due to decomposition of organic matter, perhaps this trend would reverse, given that animals (unlike some microbes) require oxygen to eat organic matter.
Chlorophyll sometimes correlates with zooplankton
Orsi and Mecum (1986)
Orsi and Mecum (1986) examined zooplankton in the Sacramento-San Joaquin delta. They found (p. 337):
The decline in overall zooplankton abundance from 1972 to 1978 was roughly paralleled by a decrease in the mean chlorophyll a concentration from 18.5 μg l-1 in 1972 to 4.5 μg l-1 in 1978. Simple linear correlation coefficients between zooplankton density and chlorophyll a concentrations for 1972-1978 were 0.864 (p < 0.05) for rotifers, 0.522 (not significant) for cladocerans, and 0.941 (p < 0.01) for copepods (Figs. 15, 16, and 17).
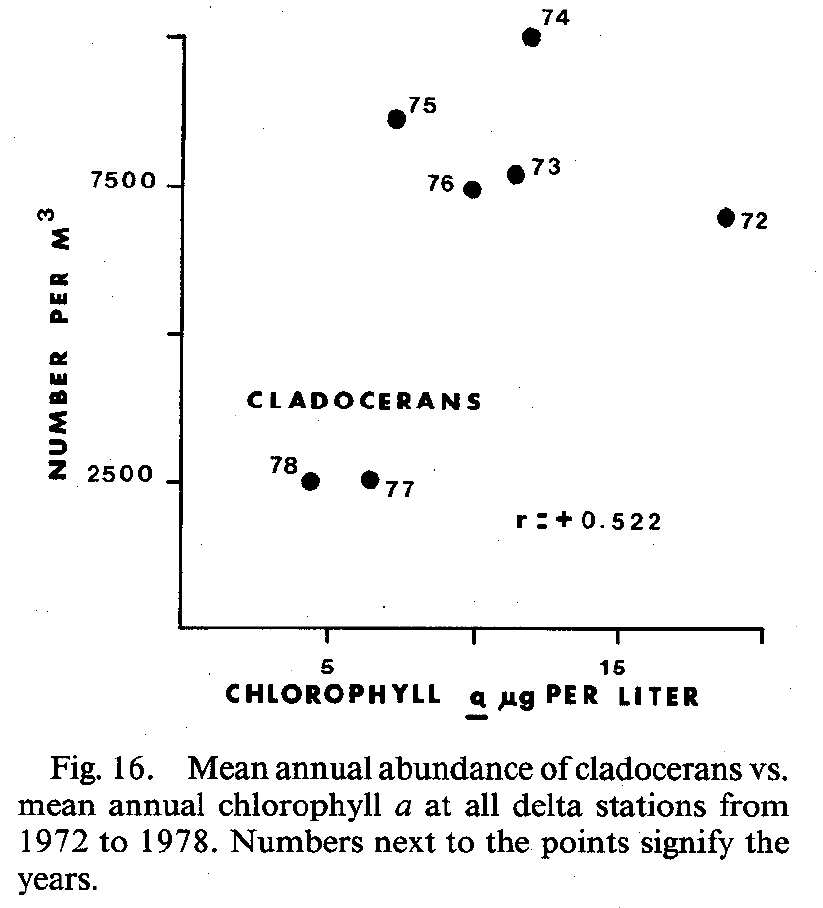
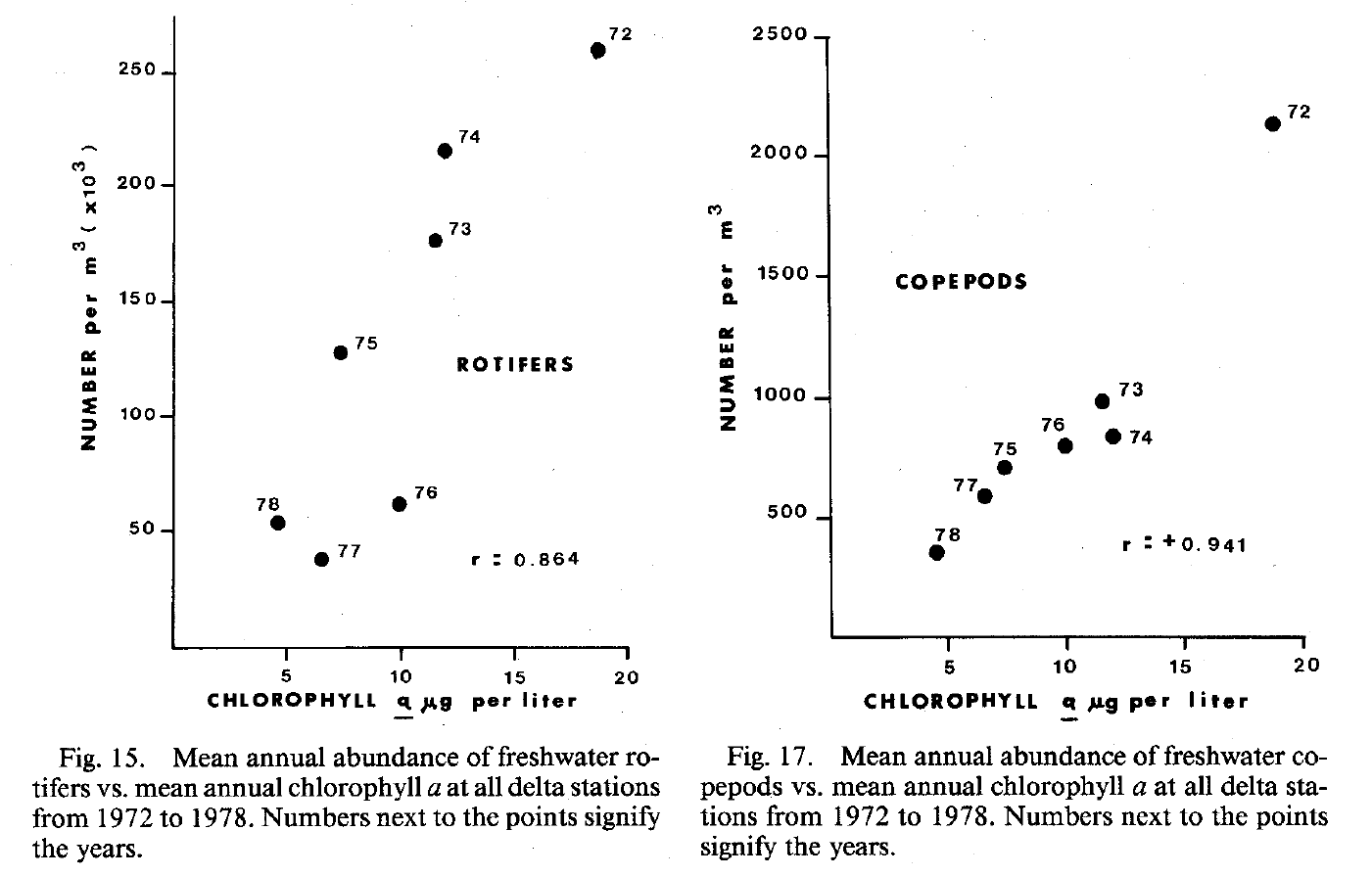
Orsi and Mecum (1986), pp. 337-38:
The statistically significant correlation between zooplankton densities and chlorophyll a concentrations agree with the result of laboratory experiments demonstrating that phytoplankton availability regulates zooplankton growth and reproduction (Weglenska 1971; Zurlini et al. 1978; Chi-Hsiang and Armitage 1980; Robertson and Salt 1980; Kankaala and Wulff 1981), and field studies in which zooplankton abundance or biomass was positively correlated with phytoplankton biomass or chlorophyll a (McCauley and Kalff 1981; Canfield and Watkins 1984).
Pace et al. (1992)
Pace et al. (1992) examined "zooplankton in the tidal freshwater portion of the Hudson River" in New York, USA (p. 1060). The authors found a correlation of Bosmina with chlorophyll, though not a correlation of cyclopoid copepods with chlorophyll (pp. 1064, 1066):
We tested whether spatial variation was related to food resources by comparing average chlorophyll concentrations with averages of zooplankton abundance across all surveys. Using average abundance values for each station removes any effect of seasonally important factors such as temperature and discharge. Patterns of spatial variation for Bosmina were strongly related to differences among stations in chlorophyll if the most seaward station (Haverstraw Bay) was not considered (Fig. 6). In the case of Haverstraw, the abundance of Bosmina was low relative to the chlorophyll concentration. This section of the river is probably near the seaward limit of Bosmina, a freshwater species. Densities of cyclopoid copepods were not significantly related to differences in chlorophyll among stations.
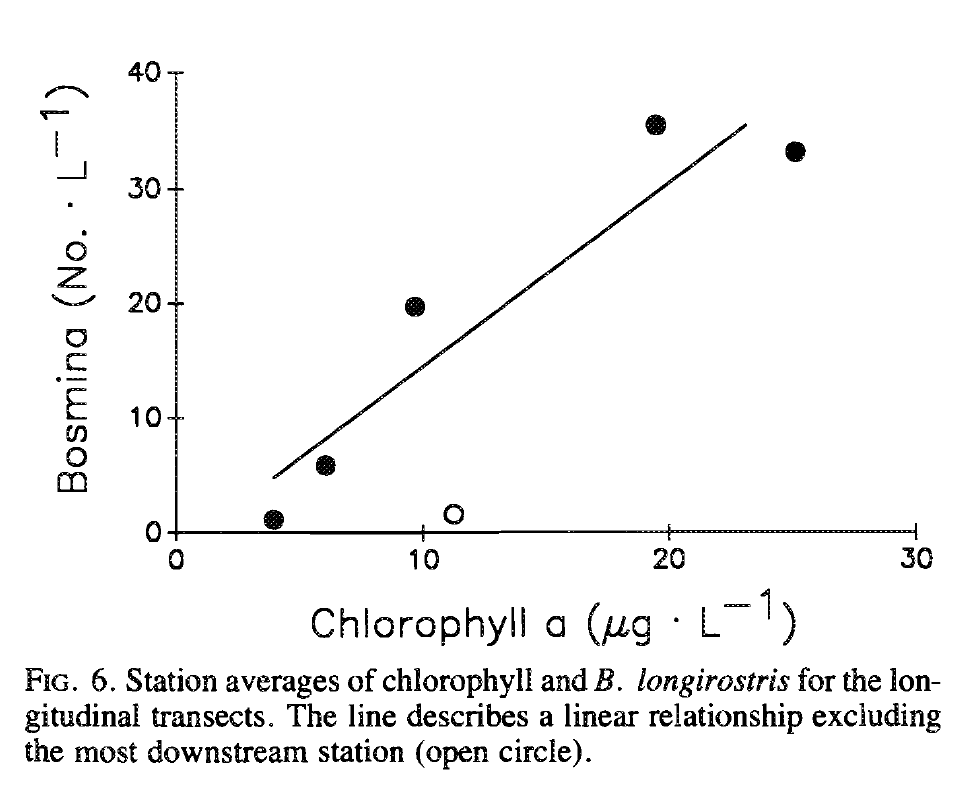
The authors also mention (p. 1066) that "It has been previously documented for lakes that zooplankton biomass varies positively as a function of algal biomass (McCauley and Kalff 1981)."
Trophic status and zooplankton
This study says in its "Introduction" that "Eutrophication caused by water pollution results in excessive propagation of zooplankton cyclops in waterbodies" (p. 1826).
Pinto-Coelho et al. (2005)
Pinto-Coelho et al. (2005) explain in their "Introduction" (p. 349):
Zooplankton have long been used as an indicator of lake trophy (Gannon and Stemberger 1978; Bays and Crisman 1982; Pejler 1983). Nonetheless, variable responses of zooplankton to trophic status are common (Ravera 1996), perhaps in part because zooplankton also respond to other environmental factors, such as lake water chemistry, shoreline disturbances, and watershed land use (Pinel-Alloul et al. 1990; Stemberger and Lazorchak 1994; Patoine et al. 2000) as well as levels of vertebrate and invertebrate predation (Hulot et al. 2000).
Pinto-Coelho et al. (2005) summarize more previous literature in the "Discussion" (p. 359):
High densities of total zooplankton (over 103 individuals·L–1) are common in tropical waters. Crustacean zooplankton, especially small cladocerans and cyclopoids, can often reach such densities in hypereutrophic lakes, reservoirs, and fertilized ponds of hatchery stations (Branco and Senna 1996). Even the small calanoid Scolodiaptomus corderoi can occur and even form large populations in the most eutrophic reservoirs of Brazil (Reid et al. 1988).
Pinto-Coelho et al. (2005) then present their findings (p. 359):
Our study confirmed that more nutrient-enriched lakes or reservoirs support greater crustacean zooplankton density and biomass. This is valid for both temperate and tropical regions. The regression models relating biomass of total crustacean zooplankton to [total phosphorus] TP were significant for most regions (excepting only Florida lakes) with R2 varying between 0.45 and 0.77. The predictive power of our large-scale regression models is similar to that of other studies (R2 = 0.63–0.86) done either at large geographic scales including 44 lakes mostly from temperate regions of North America and Europe (Hanson and Peters 1984) or at small regional scales in 16 nutrient-poor lakes in southcentral Ontario (Yan 1986) and 12 mesoeutrophic lakes in southern Quebec (Pace 1986). Patalas (1972) also found a significant regression (R2 = 0.92) between crustacean abundance and phosphorus loading in the Great Lakes.
Other information
This page reports: "Water-fowl enthusiasts (e.g. duck hunters) may want a lake to be eutrophic so that it will support a large population of waterfowl."
Eutrophication kills mayflies
Mayflies can swarm in massive numbers in some regions for about 1 day while the insects mate. Mayflies are an indicator of water quality and can be killed by eutrophication:
A famous study involving mayflies was conducted in Lake Erie during the 1950s and early 1960s. Lake Erie was badly polluted at that time, especially by organic debris associated with sewage and algal growths, the decomposition of which consumed most of the oxygen in the waters of deeper parts of the lake. The development of anoxic conditions resulted in mass die-offs of nymphs of the mayflies Hexagenia rigida and H. limbata, which were previously extremely abundant.
If eutrophication prevents trillions of mayflies from being born, this seems good.
Sunlight reaching submersed aquatic vegetation
Charles (2018) reports on the return of seagrass to the Chesapeake Bay due to reduced eutrophication: "When nutrient levels are lower, algae don't proliferate, which means the water is clearer and more sunlight can reach seagrass on the floor of the estuary." The seagrass supports a lot of animal life:
"You go down into this [seagrass] bed, and you just sit still, and you watch the animals come out," he says. "We call it the secret garden [for] all kinds of little animals and small fish and baby shellfish that spend part of their lives in this underwater forest."
In particular, seagrass plays an "incredible role" in the complicated life cycle of the blue crab, one of the bay's signature species, says Orth.
For me, the big question is whether more total animal sentience is supported by algae or by seagrass. As a proxy, one could explore whether net primary productivity is higher with algae or seagrass. My uninformed guess would be that having lots of algae causes higher productivity because more total sunlight can probably be intercepted by having a layer of algae rather than just having submersed aquatic vegetation?
Unfortunately, one of the ways of reducing nutrient pollution—"planting 'cover crops' to capture nutrients that otherwise might wash away" (Charles 2018)—plausibly increases yearly net primary productivity on farm fields.
Degree of anoxia
It's well known that severe eutrophication can kill fish due to insufficient dissolved oxygen. Is the same true for zooplankton, which also require oxygen for respiration? This source says regarding eutrophication: "The decomposition of the algae by bacteria uses up so much of the oxygen in the water that most or all of the animals die".
My intuition would be that total vertebrate and invertebrate animal populations should increase with eutrophication up to a point at which lake oxygen levels become sufficiently low, after which total animal populations would (sharply) decline. This page explains: "Oligotrophic lakes generally host very little or no aquatic vegetation and are relatively clear, while eutrophic lakes tend to host large quantities of organisms, including algal blooms. Each trophic class supports different types of fish and other organisms, as well. If the algal biomass in a lake or other water body reaches too high a concentration (say >80 TI [hypereutrophic]), massive fish die-offs may occur as decomposing biomass deoxygenates the water."
I don't recall if the studies I presented in earlier sections of this piece distinguished whether the lakes being described were oxic or anoxic. It would be helpful to look into this distinction in further research.
This source discusses gastrotrichs, which are small aquatic animals. It reports: "Kisielewski showed that gastrotrich density and species richness are positively correlated with the productivity of the habitat" (p. 165). Moreover: "Gastrotrichs are among the few animals commonly found in anaerobic environments, remaining abundant even during extended periods (months) of anoxia" (p. 165). I wonder if gastrotrichs are more numerous in anoxic eutrophic lakes than in oxic oligotrophic lakes??
If a hypereutrophic lake is only anoxic during some parts of the year, I wonder whether it still might support more total animals per year than an oligotrophic lake, because there might be lots of available organic matter during the non-anoxic times of the year? As an example using made-up numbers, suppose that an oligotrophic lake creates 5 units of food per year, all of which are available for consumption by aerobic invertebrates. Meanwhile, suppose that a hypereutrophic lake creates 100 units of food per year, of which only 10 can be decomposed aerobically because the lake is anoxic most of the time. The hypereutrophic lake would still support more total invertebrate animals over the whole year.